Bacterial endotoxin presents a risk to sterile medicine products, especially those administered intravenously or intrathecally. A component of the outer cell membrane of Gram-negative bacteria, endotoxins are known to induce a febrile response in humans and other mammals.
The endotoxin complex contains many cell wall components including, but not limited to, phospholipids, lipoproteins, and lipopolysaccharides. Lipopolysaccharide is the biologically active portion and it is pyrogenic. Bacterial endotoxin is a synonym for lipopolysaccharide1.
Sterile manufacturing processes include bioburden control measures to reduce the presence of and risk presented by Gram-negative bacteria, and process stages to remove endotoxins. These steps are supported by testing, for which the Limulus amoebocyte lysate (LAL) test is the most well-known, although not the only method available2.
Bacterial endotoxin is a synonym for lipopolysaccharide
Towards the end of the manufacturing process, at the point of fill-finish, materials and glass vials required for sterile parenteral manufacture, which can be depyrogenated by dry heat, should be depyrogenated in an oven or a tunnel.
Depyrogenation by dry heat is a quality control step. Medicines dispensed into glassware that contains levels of endotoxin above a particular threshold can cause a pyrogenic reaction or endotoxic shock to the patient. The risk becomes of even greater seriousness where medicines are for patients who are immunosuppressed.
What is depyrogenation?
The term pyrogen derived from the Greek word pyrexia. The presence of a sufficient level of pyrogens, within the bloodstream, like endotoxin, causes the release of endogenous factors triggering an immunological response within the human body; fever is the most common symptom.
The elimination of all pyrogenic substances, including bacterial endotoxin is known as depyrogenation, and it is generally achieved by complex processes of removal or inactivation.
Depyrogenation is a vital part of the manufacture of pharmaceutical products and it is distinct from sterilisation. Sterilisation does not necessarily destroy microbial by-products and toxins.
Depyrogenation by dry heat is a quality control step
Endotoxin, for example, is extremely heat stable and is not destroyed by standard sterilisation cycles such as autoclaving. Depyrogenation may be achieved through filtration, distillation, chromatography, or heat inactivation3. Furthermore, endotoxicity is not necessarily lost with the loss of viability of microorganisms. As bacterial cells are destroyed, their cellular by-products, such as lipopolysaccharide, often remain. Lipopolysaccharide is heat resistant and not affected by standard filtration or irradiation.
One important application of depyrogenation is with the treatment of glass vials and bottles used for the dispensing of sterile medicines. This form of depyrogentation is achieved through the use of depyrogenation ovens or tunnels.
The focus of depyrogenation is with bacterial endotoxin because this type of pyrogen is the most likely to be found given the amount of water used in pharmaceutical processing and the association with Gram-negative bacteria, which represent typical water system contaminants.
Depyrogenation by dry heat
Depyrogenation ovens and tunnels can be designed to both depyrogenate and sterilise glassware used for the dispensing of pharmaceutical products. The equipment uses dry heat as the depyrogenation method.
Dry heat destroys endotoxin through the physical destruction of the endotoxin molecule. Dry heat processes achieve this by convection (transfer of heat by movement of fluid or air), conduction (transfer of heat from adjacent molecules), and irradiation (emission of heat by electromagnetic radiation).
A defined endotoxin reduction rate is a correlation between time and temperature. To depyrogenate glassware, a temperature of 250°C and a holding time of 30 minutes is typically applied to achieve a reduction of endotoxin levels by a factor of 1,000. This is the most common type of depyrogenation undertaken in the pharmaceutical industry.
Sterilisation does not necessarily destroy microbial by-products and toxins
The regulatory standard for validation of an endotoxin inactivation (depyrogenation) process is that it should be capable of reducing an endotoxin challenge through 3 log10 reduction. To ensure this limit works, it is required to clean materials before dry heat depyrogenation with WFI. Otherwise, at least in theory, you could have an item contaminated with 10,000 endotoxin units (EU) entering a validated endotoxin inactivation process and still emerging with 10 EU intact and ready to contaminate your product.
Dry heat depyrogenation is a complex process and is still poorly understood with contradictory research data. The phenomenon that complicates the picture is that inactivation may approximate to Second Order chemical kinetics with a high initial rate of inactivation, then tail off to nothing. In practice, this means that at any particular depyrogenating temperature you will get some degree of inactivation in some period of time or other, but beyond that point, you will get no further inactivation by holding the material at that temperature4.
Endotoxicity is not necessarily lost with the loss of viability of microorganisms
D values for pure endotoxin (the time required to achieve one log10 reduction in activity) at 170°C are as high as 20 minutes, which would suggest that 3 log10 reductions are achievable in 60 minutes. However, due to the complexity of the kinetics, this is not necessarily true.
Oven temperatures above 180°C will inactivate endotoxin through 3 log10. However, based on the time required at 180°C, most processes use a minimum of 250°C with peak temperatures approaching 350°C commonly achieved in depyrogenation tunnels. As well as D values, Z-values can also be taken account of. This refers to the number of degrees required to change the D value by a factor of 10. Another measure used to qualify depyrogenation device is the F value, which is the time that is required to give equivalent destruction of endotoxin at a different temperature5.
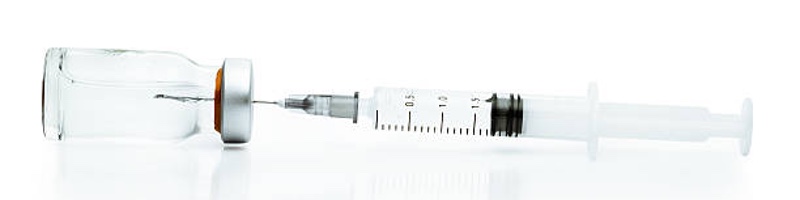
Qualifying depyrogenation tunnels
The validation of a dry heat depyrogenation process requires the evaluation of two main stages: the heating characteristics of the tunnel load and the heating cycle to be used.
Validation of the load can be determined in two ways. The first method is to determine the worst-case load, allowing the user to perform lesser loads up to the validated worse case. The second method is to validate each individual load to be used6.
Dry heat depyrogenation is still poorly understood with contradictory research data
To determine the worst-case load, the oven or tunnel performance is monitored using all load configurations. Calibrated thermistors should be used to map the ovens internal temperature profile. This step is often achieved using a configuration of two rear and two front thermistors with one central thermistor for part of the device.
As the temperature increases, the configuration of the load and the performance of the device will result in parts of the over warming quicker than others. This differential heating results in cold spots that are identified by the thermistors.
Once these are known, the time it takes for the cold spot to reach the required temperature should be determined. This equals the ramp-up time and is the minimum time the oven should be run before the timing of the depyrogenation cycle.
Calibrated thermistors should be used to map the ovens internal temperature profile
As the cold spot is the last point in the device to reach temperature, the other areas in the device will reach the required temperature ahead of the point that the timing for depyrogenation will begin. During the ramp-up, depyrogenation will already be occurring for these parts of the oven load.
As most depyrogenation temperatures are much higher than is necessary for depyrogenation the whole load will be undergoing depyrogenation before the start of the timed cycle. This provides for a substantial safety margin in the process.
Having established the worst-case load, and understanding the required ramp-up time for this load, the final part of the validation can be completed. This phase consists of seeding articles from the load to be depyrogenated with endotoxin. This involves adding endotoxin in excess of 10,000 EU onto the validated load articles. The aim is to show a least a three log10 reduction in endotoxin.
The use of positive controls is important to provide the initial starting concentration of endotoxin in the challenge articles.
The use of positive controls is important
Challenged vials should be placed throughout the device including the cold spots identified in the first part of the validation. All these controls should then be assayed.
Using the results from both the positive controls and those run through the cycle, the reduction in endotoxin achieved by the process can be calculated. It is important to use the actual concentration of the positive controls as the starting concentration. This step in itself is not easy as the amount of endotoxin you dry on an item (depending on the material of the item) may not be the maximum amount you can recover.
If the appropriate reduction is achieved, then this load should be used in combination of cycle time and temperature to ensure articles are suitably depyrogenated.
N.B. This article is featured in the March 2020 issue of Cleanroom Technology. Subscribe today and get your print copy!
The latest digital edition is available online.
References
1. Dinarello, C. (2004). Infection, fever, and exogenous and endogenous pyrogens: some concepts have changed. J. Endotoxin Res. 10, 201-222
2. Levin, J. (2019) Discovery and Early Development of the Limulus Test. In Williams, K. L. (Ed.) Endotoxin Detection and Control in Pharma, Limulus, and Mammalian Systems, Springer, USA, pp 3-16
3. Tours, N. and Sandle, T. (2008) Comparison of dry-heat depyrogenation using three different types of Gram-negative bacterial endotoxin, European Journal of Parenteral and Pharmaceutical Sciences, 13 (91): 17-20
4. Sandle, T. (2007) Some Considerations on the Use of Endotoxin Indicators in Depyrogenation Studies, Pharmaceutical Microbiology Forum Newsletter, 13 (10): 2-11
5. Cooper, J.F. and Williams, K. (2007) Pyrogenicity Case Studies, in Williams, K.(Ed). Endotoxins: Pyrogens, LAL Testing and Depyrogenation, New York: Informa Healthcare
6. Sandle, T. (2011): A Practical Approach to Depyrogenation Studies using Bacterial Endotoxin, Journal of GXP Compliance, 15 (4): 90-96
Pharmig is heading to Europe
Latest Updates & Hot Topics in Pharmaceutical Microbiology
Pharmig will be running its first European Conference on the 17-18 June in Amsterdam.
The programme is currently in the development stage but we have already secured the following speakers:
Tim Sandle – Microbiological culture media: use and application in healthcare and pharmaceuticals
David Keen – Aseptic manufacturing: The open flaw in using humans in your critical operations
Kim Morwood – Wipe efficacy test methods and opportunities for development
Willy Verstraete – Microbial biomass production in a multitude of novel contexts
Tim Sandle – Fungal contamination in pharmaceutical products and cleanroom risk
Donald Singer – Current activities of the USP Microbiology Expert Committee
Please do visit the Pharmig website for more information – www.pharmig.org.uk or contact Pharmig on info@pharmig.org.uk